When an ultra-intense laser interacts with a volume of gas it can create a plasma wave structure capable of sustaining extremely large electric fields (TV/m) over a very small spatial scale (~1 mm). Such fields are 100-1000 times greater than that achievable with a conventional accelerator, making laser wakefield acceleration (LWFA) extremely competitive in terms of cost and space compared to much larger facilities.
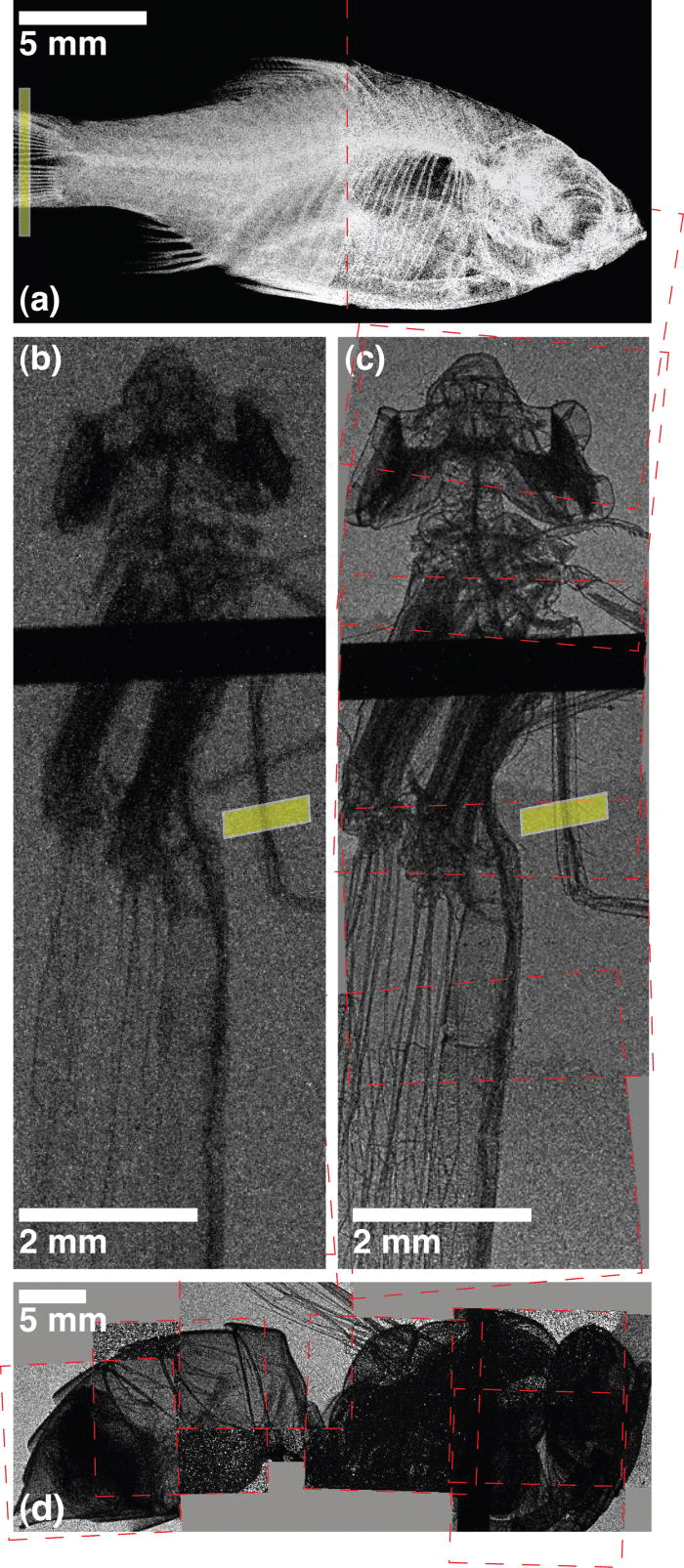 |
X-ray absorption contrast image of (a) an orange tetra fish and (b) a damselfly and x-ray phase contrast image of (c) a damselfly and (d) a yellow jacket. Images are taken with betatron radiation from a laser wakefield accelerator. (Credit – Applied Physics Letters 2011)
|
Experiments over the last 15 years at the CLF have seen many breakthroughs with LWFA, with one significant 2004 publication from an experiment on the Astra laser, that demonstrated the production of monoenergetic electron beams with an energy of ~80 MeV, now cited over 1000 times
[1]. As control of laser and gas parameters improved, electron energies soon passed the 1 GeV barrier, firstly with the use of a capillary discharge waveguide
[2] then with a gas jet medium
[3].These results opened up new applications for LWFA including injectors for conventional accelerators, or x-ray and gamma ray sources.
Betatron sources and Phase contrast imaging
Strong plasma waves that are generated when an ultra-intense laser interacts with a gaseous medium also produce transverse electric fields that introduce sufficient acceleration to the electrons within the plasma channel to produce synchrotron radiation, much like a conventional accelerator [4]. The resulting highly directional, µm-sized source of x-rays has an extremely large peak brightness owing to the quantity of x-rays produced, and the very short pulse duration (~40 fs).
One of the most exciting aspects of this table-top method of x-ray generation is that the resulting source is spatially coherent, making it ideal for performing advanced phase contrast imaging. This technique can be used when an object displays little absorption contrast (e.g. a single cell or an insect)
[5].
References
[1] Mangles et al. Nat. 431, 535 (2004)
[2] Leemans et al. Nature Phys. 2 696–9 (2006)
[3] Kneip et al. Phys. Rev. Lett. 103 035002 (2009)
[4] Kneip et al. Nat. Phys. 6, 980-983 (2010)
[5] Kneip et al. Appl. Phys. Lett. 99, 093701 (2011)
Recent CLF Results
Excitation and Control of Plasma Wakefields by Multiple Laser Pulses - J Cowley et al., Phys. Rev. Lett. 119 (2017)
The Astra laser has been used to demonstrate resonant excitation of plasma waves using a train of laser pulses. Using low energy (10s mJ), short pulse (50 fs) laser pulses wakefields have been driven using multiple pulses rather than a single, higher intensity pulse. This method could lead to higher efficiency electron acceleration that could operate at much higher repetition rates.
Laser-wakefield accelerators as hard x-ray sources for 3D medical imaging of human bone (link opens in a new window) - J Cole et al.
Scientific Reports 5 13244 (2015)
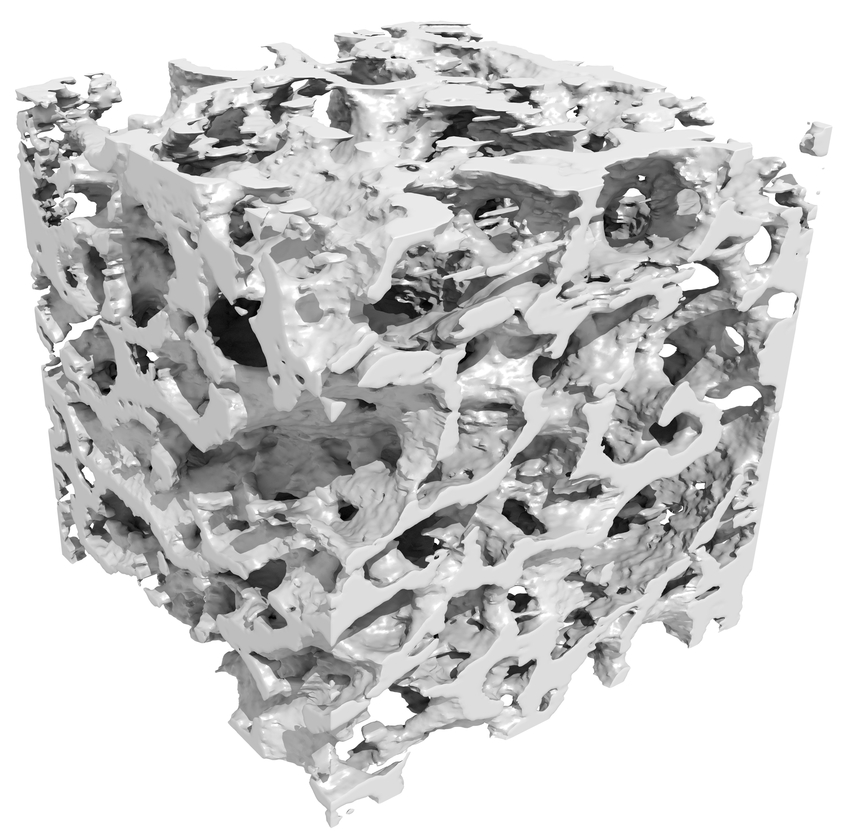
BoneCube - 3D reconstruction of a sample of human hip bone created using a laser wakefield accelerator (Credit: STFC)
X-rays from a laser-wakefield accelerator have been used to create high resolution images of dense bone material on the Gemini laser. The novel x-ray source was used to create a 3D image with a spatial resolution of around 50 microns.
Generation of neutral and high-density electron–positron pair plasmas in the laboratory (link opens in a new window) - G Sarri et al.
Nat. Comms.6 6747 (2015)
Using a compact laser-driven setup, ion-free electron–positron plasmas with unique characteristics can be produced (Credit: Nature Comms)
A group from Queen’s University Belfast recently used the Gemini laser to generate a neutral electron-positron plasma by directing a laser-accelerated electron beam into a lead block. The small divergence of the resulting plasma together with its charge neutrality will permit unique studies into a novel state of matter.
Investigation of GeV-scale electron acceleration in a gas-filled capillary discharge waveguide (link opens in a new window)P Walker et al.
New Journal Physics 15 45024 (2013)